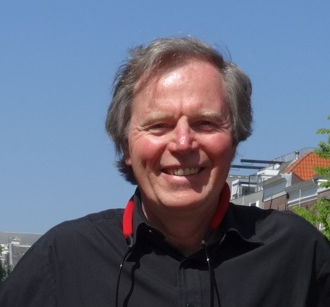
Professor Emeritus of Physics Dan Stinebring is a founding member of the North American Nanohertz Observatory for Gravitational Waves, which is an international collaboration. The NANOGrav research team, on which Stinebring is a senior researcher, published their findings on the use of radio telescopes that offer the first evidence of gravitational waves at very low frequencies. This research was cited in The New York Times, The Washington Post, The Guardian, NPR, and Science.
This interview has been edited for length and clarity.
Can you explain the discoveries your team made?
What we’re doing is searching for, and what we believe we have evidence of, is gravitational waves in the universe. First, let’s talk about what a gravitational wave is. A gravitational wave is analogous to a light wave, but instead of being electromagnetic, it’s related to the deformation of spacetime itself. We live in spacetime, and we think of it as almost like a membrane, like a rubber sheet. If you were to push down quickly on one side of it, a wave would propagate outward along this rubber sheet at the speed of light. A gravitational wave is a propagation of a deformation of spacetime that spreads outward or propagates outward at the speed of light. It was predicted by Einstein in his theory of gravity. Einstein predicted gravitational waves in 1916, and they were confirmed to exist in 2015 by the LIGO experiment, which detected gravitational waves from two merging black holes.
What we are doing — and we in this case are several hundred scientists around the world — is trying to detect gravitational waves, but in a much different wavelength regime. Just like the electromagnetic spectrum has a whole range of phenomena along it, we believe that there’s also a gravitational wave spectrum out there as well as the short wavelength gravitational waves that the LIGO collaboration detected.
Now, we’re trying to detect gravitational waves, but in a different wavelength regime, similar to how the electromagnetic spectrum has various wavelengths. We believe there are long-wavelength gravitational waves with wavelengths of light-years. That’s trillions of years in length. These waves are passing through us right now, slightly deforming and stretching us as they go by. So it’s all pretty phenomenal.
What are you trying to detect within your research?
We’re looking for a multitude of supermassive black hole systems in distant galaxies. These black holes, each a billion times the mass of the sun, orbit each other, creating gravitational waves. Our experiment involves hundreds of scientists worldwide.
Gravitational waves squeeze and stretch spacetime as they pass by. Imagine the Earth with a gravitational wave coming from above. During one half of the wave’s cycle, it pushes in, and during the other half, it stretches out.
Gravitational waves cause Earth to stretch and compress periodically, affecting the timing of pulses from pulsars. Pulsars are rapidly rotating neutron stars that emit beams of radio waves, much like a lighthouse. We use them as precise celestial clocks because their pulses are incredibly regular.
When gravitational waves affect Earth, they cause pulses from different pulsars to arrive either slightly early or slightly late, depending on their position relative to each other. If two pulsars are 90 degrees apart in the sky, one will be early while the other is late, creating an anticorrelation. If they are in the same part of the sky, there will be a correlation between their pulse timings.
By observing these timing correlations or anticorrelations among pulsars, we can indirectly detect gravitational waves. This is the essence of our experiment, and it involves many pulsars, each acting as a precise gravitational wave detector.
What drew you to studying gravitational waves?
It was the pulsars. Initially, a group of us were drawn together because we loved pulsars. We were incredibly impressed by what pulsars could achieve in terms of high precision science. High precision is really crucial here. Over time, as the collaboration grew, we brought in people from the gravitational wave community because that was the natural way to expand. These were people who didn’t know anything about pulsars or radio telescopes, but they knew about gravitational waves and how to detect them, and they were well-versed in sophisticated data analysis techniques that we were not using at the time. I’ve truly fallen in love with gravity and some of the amazing things we’ve been studying. It’s been a real pleasure for me.
News coverage has described this as a major discovery. Can you explain a little bit about why this is important?
Gravity is one of the four fundamental forces of nature. And in some sense, it’s the most familiar. We all experience it constantly. But there are also deep puzzles connected to gravity. But let me go in a slightly different direction, in terms of this being fundamental research in science. We’re constantly testing our theories, right? We have this beautiful edifice of Einstein’s theory of gravity: his theory of general relativity. And it’s been tested in numerous, numerous ways.
But, as I mentioned, there are deep puzzles within physics. In particular, how does gravity connect with quantum mechanics, our fundamental theory of the super small? The theories are radically different from each other. There’s no natural connection point between them. And this has been recognized as a fundamental problem in physics for at least 50 years. And so perhaps there’s something incomplete about Einstein’s theory of gravity. There’s something missing. Is it possible that through our observations of celestial gravitational waves we could find some deviations of Einstein’s theory of gravity and find some fundamental reworking making it more complete? That’s one possibility. Another aspect that makes this work very exciting and I think very fundamental, is that we don’t understand our universe, and in particular, we don’t completely understand the earliest stages of our universe, when galaxies were just forming.
You were mentioning how you think that this research has a big future. Can you tell us what you think people would do with this research?
I fully expect that this experiment will continue for the next 30 to 50 to 100 years. And over 30 to 50 to 100 years, or even just within the next five years, we will be detecting an individual supermassive black hole pair. Another area that we’re expecting to go again over five, 10, 50 years is more fully characterizing what this background of gravitational waves is. Is it the same from all directions in the sky?
How has working at Oberlin contributed to your research?
This research has been tremendously exciting for Oberlin students through the years. I tallied it up, and since 2007, there have been 48 Oberlin students who have worked on this research with me. We have had a blast, and we’ve gone to telescopes like the Arecibo Observatory and the Green Bank Telescope in West Virginia. We’ve gone to conferences in the U.S. and around the world to talk about gravitational waves, pulsars, and this particular specialty we have at Oberlin.